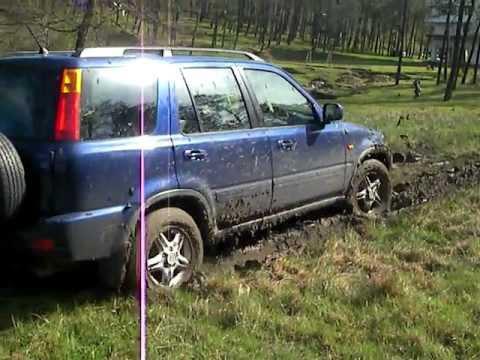
CD19-Targeted CAR T Cells as Novel Cancer Immunotherapy for Relapsed or Refractory B-Cell Acute Lymphoblastic Leukemia – Hematology – Oncology
CD19-Targeted CAR T Cells as Novel Cancer Immunotherapy for Relapsed or Refractory B-Cell Acute Lymphoblastic Leukemia
Clinical Advances in Hematology & Oncology
October 2016, Volume 14, Issue Ten
Marco L. Davila, MD, PhD, and Renier J. Brentjens, MD, PhD
Dr Davila is an associate member in the Department of Blood and Marrow Transplantation and the medical director of the Cell Transfer Facility, both at the H. Lee Moffitt Cancer Center and Research Institute in Tampa, Florida. Dr Brentjens is the director of Cellular Therapeutics and an associate member in the Leukemia Service at Memorial Sloan Kettering Cancer Center in Fresh York, Fresh York.
Marco L. Davila, MD, PhD
Department of Blood and Marrow Transplantation
H. Lee Moffitt Cancer Center and Research Institute
twelve thousand nine hundred two Magnolia Drive
Tel: (813) 745-7202
Fax: (813) 745-1328
Abstract: Immunotherapy has demonstrated significant potential for the treatment of patients with chemotherapy-resistant hematologic malignancies and solid tumors. One type of immunotherapy involves the adoptive transfer of T cells that have been genetically modified with a chimeric antigen receptor (CAR) to target a tumor. These hybrid proteins are composed of the antigen-binding domains of an antibody fused to T-cell receptor signaling machinery. CAR T cells that target CD19 recently have made the hop from the laboratory to the clinic, and the results have been remarkable. CD19-targeted CAR T cells have induced finish remissions of disease in up to 90% of patients with relapsed or refractory B-cell acute lymphoblastic leukemia (B-ALL), who have an expected accomplish response rate of 30% in response to chemotherapy. The high efficacy of CAR T cells in B-ALL suggests that regulatory approval of this therapy for this routinely fatal leukemia is on the horizon. We review the preclinical development of CAR T cells and their early clinical application for lymphoma. We also provide a comprehensive analysis of the use of CAR T cells in patients with B-ALL. In addition, we discuss the unique toxicities associated with this therapy and the management schemes that have been developed.
Immunotherapy for cancer has generated significant excitement owing to unprecedented responses in patients with chemotherapy-refractory acute leukemia and solid tumors. The mechanism of act for most immunotherapy includes the activation of a T-cell response against a malignancy. Cancer spread can be mediated by blocking T-cell suppression signals or by redirecting a T cell to a tumor target with an antibody specific to both T cells and tumors. In the case of adoptive T-cell therapies, a patient’s own T cells are isolated and manipulated in the laboratory and then reinfused. The two main types of adoptive T-cell therapies employ either tumor-infiltrating lymphocytes (TILs) or chimeric antigen receptor (CAR) modified T cells.
In TIL therapy, TILs are isolated from solid tumors and expanded over several weeks to months in a laboratory to generate a sufficient number of tumor-reactive T cells. One Some patients with metastatic tumors practice durable finish remission (CR), which is not possible with salvage chemotherapy. Two
One of the major disadvantages of TIL therapy is the culture time required to generate a sufficient number of TILs to mediate treatment responses. Albeit TIL production has evolved from taking several months to taking several weeks, antigen stimulation and culture with interleukin two (IL-2) commonly results in terminal-differentiated T cells with limited in vivo persistence. This may explain why most patients have no antitumor effect from TIL therapy. Three Also, requiring patients with refractory disease to wait for TIL infusion is problematic for those who are very ill.
Engineering T cells to express CARs overcomes this time disadvantage, and includes several other advantages over TIL therapy. The CAR is a hybrid protein that includes an antigen-binding domain, derived from an antibody, fused to a transmembrane domain followed by T-cell activation domains associated with the T-cell receptor (TCR). Four A T cell modified with a CAR is talented with a fresh antigen specificity, and strapping its antigen supports T-cell activation and killing of the target cell. With sturdy gene-transfer technologies available for human T cells, a sufficient number of tumor-reactive T cells can be produced in as little as one week. Five Also, CARs are universal antigen receptors that can be used in all patients owing to their antigen-binding domains being derived from antibodies. In contrast, TIL therapy and other T-cell therapies are human leukocyte antigen (HLA)-restricted, so they recognize tumor-specific antigen when it is introduced by certain major histocompatibility sophisticated (MHC) molecules. Furthermore, TCRs recognize brief peptide sequences as tumor antigens, whereas CARs can recognize proteins, lipids, and/or carbohydrates as antigens. Ultimately, owing to the modular nature of the CAR, it can be continually modified and refined for optimization, or potentially to develop fresh functions.
Zelig Eshhar developed the very first CAR using what he called a T-body treatment. He combined a single-chain variable fragment (scFv), recreating an antigen-binding domain, with TCR-associated activation domains from CD3ζ or CD3ϒ. Six Eshhar and colleagues validated the function of genetic-retargeted CAR T cells in vitro. Several groups later confirmed this by developing CARs against various tumor targets in vitro. Seven However, these first-generation CARs displayed limited persistence and poor tumor control in vivo. Eight Poor efficacy in mice prevented the clinical translation of this technology to patients. However, CAR T-cell investigators were able to overcome this in vivo inefficacy by engineering CAR T-cell activation to mimic more closely physiologic T-cell activation. The 2-signal rule of T-cell activation states that TCR activation via CD3ζ is insufficient for accomplish activation and long-term T-cell persistence. Instead, a costimulatory signal, such as CD28, is required as signal Two. Modification of CARs to include both CD3ζ and CD28 resulted in similar in vitro function, but also supported sturdy tumor killing and long-term CAR T-cell persistence in vivo. 9-12 Albeit second-generation CARs paired CD28 with CD3ζ, researchers ultimately demonstrated that other costimulatory agents, such as OX40, 41BB, or CD27, could similarly enhance CAR T-cell function in vivo. 13,14 Third-generation CARs combining two costimulatory domains with CD3 also have been developed, but have not been clinically evaluated to the extent of second-generation CARs. This preclinical validation of CAR T cells supported clinical evaluation in early-phase clinical trials. Owing to the latest number of reports from numerous groups detailing outcomes of infusing CD19-targeted CAR T cells in almost one hundred patients with B-cell acute lymphoblastic leukemia (B-ALL), our review concentrates on this disease. However, because early trials targeting non-Hodgkin lymphoma (NHL) with CD19-targeted CAR T cells provided significant insights and set the foundation for the good success in treating B-ALL, the review embarks by highlighting these efforts.
Clinical Evaluation of CD19-Targeted CAR T Cells for B-Cell Malignancies
Early Application of CAR T Cells in NHL and Chronic Lymphocytic Leukemia
The very first trials evaluating CAR T cells for B-cell malignancies focused on NHL, and targeted CD19 or CD20 antigens. One of the most significant observations came from a group at the Baylor College of Medicine, whose work confirmed the results of preclinical studies comparing first-generation and second-generation CAR T cells. Savoldo and colleagues fifteen infused patients with NHL with a combination of T cells that were modified with either a first-generation CD19-specific CAR or a second-generation CD19-specific CAR that contained a CD28 costimulatory domain. They demonstrated that the second-generation CAR T cells expanded better and persisted longer than the first-generation CAR T cells, albeit responses were modest. In addition, investigators at the Memorial Sloan Kettering Cancer Center (MSKCC) demonstrated that lymphodepletion with conditioning chemotherapy was required for optimal CAR T-cell function. Brentjens and colleagues sixteen treated patients with T cells modified with a second-generation CAR that included a CD28 costimulatory domain. The very first cohort was infused with CD19-targeted CAR T cells alone, whereas the 2nd cohort was treated with cyclophosphamide conditioning chemotherapy (1.Five to Three.0 g/m two ) prior to CAR T-cell infusion. They demonstrated that expansion, persistence, and responses were improved in the conditioned cohort. 16
Albeit these early trials and others established the safety of CD19-targeted CAR T cells, they lacked visible evidence of T-cell mediated eradication of disease. However, a case report from the National Cancer Institute (NCI) that used a second-generation CAR with CD28, and a case series from the University of Pennsylvania (UPENN) that used a second-generation CAR with 41BB, established proof of principle that CAR T cells are a potent and targeted immunotherapy. 17-19 Both groups demonstrated rapid resolution of disease shortly after infusion with the CD19-targeted CAR T cells. However, what clearly established the role of the CAR T cells in mediating the treatment response was a concomitant B-cell aplasia observed in some patients that lasted longer than one year. 17-19 Such a long-term B-cell aplasia could not be attributed to chemotherapy, but was predicted as an on-target, off-tumor toxicity of CD19-targeted CAR T cells. In addition, these case reports also detailed a unique set of toxicities, including fevers, hypotension, and hypoxia, that were the very first hallmarks of an inflammatory response that we now recognize as cytokine release syndrome (CRS). These anecdotal results have been followed up with trials optimizing conditioning chemotherapy and focusing on certain NHL subtypes, which has resulted in objective response rates of up to 53% for patients with chemotherapy-refractory NHL. 20-22 More importantly, they provided the framework for trials evaluating CD19-targeted CAR T cells for relapsed or refractory B-ALL that likely will switch the standard of care for this poor-outcome disease.
Application of CD19-Targeted CAR T Cells for Relapsed/Refractory B-ALL
In the last three years, six reports have detailed trials of CD19-targeted CAR T cells for patients with B-ALL. 23-28 The results have been uniformly remarkable, despite a myriad of trial differences that include a broad spectrum of ages treated, CAR T-cell production systems that were either lentiviral or gammaretroviral, and different costimulatory domains in the CAR (CD28 or 41BB). The very first reports came from the MSKCC group and detailed outcomes from treating adults (n=16) with relapsed or refractory B-ALL. 23,24 This was followed by reports from investigators at UPENN, 25,26 who treated children and youthfull adults (n=25) and adults (n=Five), and the NCI, who reported their efforts with treating children and youthfull adults (n=21). Twenty seven Most recently, the group at the Fred Hutchinson Cancer Research Center (FHCRC) reported their outcomes after treating thirty adults. Twenty eight Albeit all groups utilized second-generation CD19-targeted CAR designs, the differences included scFv and costimulatory domains. The UPENN, NCI, and FHCRC groups developed an scFv from the FMC63 hybridoma, and the MSKCC developed their scFv from the SJ25C1 hybridoma. 29,30 In addition, the CARs from the MSKCC and NCI groups utilized the same CD28 transmembrane and costimulatory domain paired to the CD3ζ intracellular activation domain, whereas those from the UPENN and FHCRC groups utilized the 41BB costimulatory domain paired to CD3ζ. 13,28,30,31
The UPENN and FHCRC groups produced their CD19-targeted CAR T cells via lentiviral gene transfer, whereas the NCI and MSKCC groups used gammaretrovirus. Both viral technologies appeared equivalent, with efficient gene transfer and minimal production failures of required doses (MSKCC, 1; UPENN, unknown; NCI, Two; FHCRC, 0, but one unsuccessful CD8 enrichment). 23-28 Considering the results with NHL, all trials required lymphodepleting chemotherapy before CAR T-cell infusion, which included cyclophosphamide (MSKCC), physician’s choice (UPENN), and fludarabine (FLU) plus cyclophosphamide (CY; the NCI). Albeit no visible differences occurred that could be attributed to the different conditioning regimens used across these trials, the FHCRC group demonstrated that FLU/CY vs CY alone or with etoposide enlargened CAR T-cell persistence and expansion, and more importantly, disease-free survival. CAR T-cell doses ranged from two × ten Five /kg up to two × ten 7 /kg, with MSKCC having a fixed-dose trial (Three × ten 6 /kg) and the NCI and FHCRC groups having dose-escalation trials (1 × ten 6 /kg followed by three × ten 6 /kg, and two × ten Five /kg followed by two × ten 6 /kg and two × ten 7 /kg, respectively). 23-28 The maximum tolerated doses determined by the NCI and FHCRC groups were one × ten 6 /kg and two × ten 6 /kg, respectively. There was also a difference in cell formulation for infusion. Most probe sites infused bulk CAR T cells after production, however, the FHCRC group very first isolated CD8 central memory and CD4 T cells separately before CAR T cell production. 23-28 Afterward, they formulated the product to be composed of an equivalent number of CD4 and CD8 CAR T cells before adoptive transfer into the patient. The rationale for derivation of the CAR T-cell product from CD8 central memory cells was from preclinical work that demonstrated this selected composition provided superior control of leukemia. 32
Despite the differences inbetween these trials for relapsed or refractory B-ALL, the outcomes and toxicities were similar. The CR rates were 88% (MSKCC), 90% (UPENN), 67% (NCI), and 90% (FHCRC). 23-28 In comparison, the expected CR rate for relapsed or refractory B-ALL treated with salvage chemotherapy is 30%. Even blinatumomab (Blincyto, Amgen), which recently was approved for this indication, had a CR rate of only 43%. 33,34 Furthermore, these CRs were high-quality molecular remissions, as suggested by lack of minimal residual disease when evaluated with high-sensitivity assays such as flow cytometry, quantitative polymerase chain reaction, and/or deep sequencing for immunoglobulin H rearrangements. The molecular CR rate ranged from 60% to 90%. 23-28 Consistent with the high quality of these remissions is their durability. The UPENN group reported that at six months, the probability of event-free survival was 67% and the probability of overall survival was 78%. The NCI group reported that overall survival was 52% at ten months, and the FHCRC group reported that disease-free survival was greater than 60% at a median follow-up of three hundred days. 25-28
The most evident difference detected inbetween the correlative studies was the persistence of the CAR T cells. The CAR T cells from the NCI and MSKCC groups did not persist beyond two to three months from adoptive transfer, whereas the CAR T cells from the UPENN and FHCRC groups from time to time could be detected beyond six months. This difference may be related to the discrete biologic functions encoded by the costimulatory domains. Latest reports suggest that CAR T cells with CD28 drive more rapid expansion and effector-like functions, whereas 41BB drives more memory T-cell functions. 35,36 Regardless of the differences in CAR T-cell persistence, they do not emerge to result in different efficacies because the initial remission rates are equivalent and, albeit with limited follow-up, the durability of remissions is similar as well. This suggests that sturdy CD28-mediated CAR T-cell expansion is sufficient to induce high-quality molecular remissions in a brief period. Indeed, we (Drs Davila and Brentjens) were able to detect bone marrow molecular CR within as little as eight days after adoptive transfer, whereas initial reports from UPENN suggested the 41BB-containing CAR required longer to induce a molecular CR. 23,26
Despite these extraordinaire response rates, follow-up has been long enough to detect some relapses. Early relapses were related to prolonged (eg, one month) corticosteroid administration, which resulted in decreased CAR T-cell expansion. Twenty three Similar relapses have not been ascribed yet to brief pulses of corticosteroids. Other relapses show up to be related to immune escape. For example, out of the seven relapses reported by the UPENN group, three had evidence of a CD19-negative immunophenotype. Twenty five Similarly, the FHCRC group reported nine relapses, albeit all but two patients were given conditioning chemotherapy that lacked fludarabine. Two of these nine relapses were categorized as CD19-negative ALL tumors, and two CD19-negative relapses were detected by the NCI group. 27,28 It has been reported that some of the CD19-negative relapses were due to alternative splicing of CD19 exons that liquidated the epitope recognized by the CAR, albeit it is possible that the entire CD19 protein also may be downregulated. Thirty seven However, CD19-negative acute leukemia relapses after CD19 CAR T-cell infusion also have been detected with a myeloid immunophenotype. Thirty eight Based on genetic analysis of the leukemia before and after CAR T-cell treatment, it emerges the immune escape was due to selective outgrowth of a pre-existing CD19-negative myeloid clone, or possibly a retro-differentiation of a B-ALL to a myeloid leukemia.
CAR T Cell–Associated Toxicities
Reinduction of CRs was associated with a unique set of clinical signs and symptoms of a massive inflammatory disorder. Shortly after infusion of CAR T cells, patients developed high-grade fevers that progressed, along with hypotension and respiratory distress. A large increase in cytokines coincided with these toxicities, so this disorder has been classified as CRS. 23-28 It is likely that the CRS is related to the widespread activation of a large number of tumor-specific T cells, considering that similar toxicities have been reported with blinatumomab and anti-CD28 antibodies. 39,40 Albeit some cytokines, such as interferon-γ, IL-6, and IL-10, are commonly enhanced after CAR T-cell infusion, there is no consistent pattern of cytokine upregulation from patient to patient, which is most likely due to the individualized nature of the CAR T-cell therapy. The CRS can become severe and require intensive medical management, which occurred at all trial sites. Grading schemes have been developed to identify CRS that requires aggressive monitoring and interventions. The MSKCC group identified severe CRS based on the presence of fevers, cytokine elevations, and clinical signs of severe cytotoxicity, such as hypotension requiring vasopressor agents or hypoxia requiring mechanical ventilation. Twenty three A collaborative group of CAR T-cell investigators developed a revised scheme based on the requirement of medical interventions to support patients; grade one is self-limiting, whereas grade four is life-threatening. Forty one Comparison of the grading schemes suggests that grades three to five by the criteria of Lee and colleagues forty one would be classified as severe CRS by the criteria of Davila and colleagues. 23
Severe CRS was reported by the MSKCC group in seven out of sixteen patients, by the UPENN group in eight out of thirty patients, by the NCI group in six out of twenty one patients, and by the FHCRC group in seven out of thirty patients. 23-28 CRS has even resulted in fatal toxicities, albeit this has been infrequent. Only two deaths out of ninety seven B-ALL patients treated with CD19-targeted CAR T cells were attributed to CRS. 23-28 For most patients, mild to moderate CRS (ie, grades 1-2) is self-limiting and requires supportive care alone, but in severe cases medical intervention is required. Cytokine-directed therapy and corticosteroids are the mainstay of CRS medical management. Cytokine-directed therapy includes tocilizumab, which inhibits IL-6 receptor signaling, and etanercept (Enbrel, Amgen), which inhibits tumor necrosis factor signaling. Tocilizumab is more widely employed because IL-6 commonly increases during the intensification of CRS. Tocilizumab rapidly ameliorates the CRS without any reported deleterious effects on CAR T-cell expansion or persistence, or durability of remissions.
The MSKCC group treated three patients with tocilizumab (Actemra, Genentech), two with corticosteroids, and one with both tocilizumab and corticosteroids; the UPENN group treated nine patients with tocilizumab and six with corticosteroids, the NCI group treated two patients with tocilizumab and two with both corticosteroids and tocilizumab, and the FHCRC group treated seven patients with tocilizumab and three with corticosteroids. 23-28 There were reports that corticosteroids may inhibit CAR T-cell expansion and reduce the durability of remissions, but this was likely due to prolonged administration. Nineteen,23,24 Considering that corticosteroids rapidly reduce cytokines and eliminate fevers, shorter pulses should attenuate the CRS without affecting CAR T-cell expansion and function.
Clinical investigators have evaluated whether laboratory markers could be used to predict patients who will have severe CRS. The MSKCC group determined that leukemia cargo strongly predicted which patients have severe CRS. Twenty three All but one of the patients with morphologic residual leukemia (marrow blasts ≥5%) developed CRS, whereas none of the patients with MRD or in molecular CR had severe CRS. This observation has been confirmed by all the other CAR T-cell studies of B-ALL. The MSKCC group also determined that C-reactive protein could be monitored daily and used to identify patients who would shortly develop severe CRS toxicities. Twenty three All the CD19 CAR T-cell studies have confirmed that elevation of certain cytokines, such as IL-6 and interferon-γ, strongly correlate with severity of CRS. 23-28 The NCI group also demonstrated that CAR T-cell expansion correlated with CRS severity. Twenty seven Based on clinical practice with CD19-targeted CAR T cells, management schemes for CRS have been developed. The guidelines from Lee and colleagues forty one reserve tocilizumab and/or corticosteroids for patients with severe CRS (grade ≥3) or patients with moderate CRS (grade Two) who have comorbidities. The guidelines from Davila and colleagues twenty three similarly recommend tocilizumab and/or corticosteroids for severe CRS, but also include tumor cargo as a branch point for management. Based on the extensive data correlating CRS severity with leukemia cargo, patients with MRD can be infused and potentially discharged with minimal follow-up because their probability of CRS toxicity is very low.
There is also evidence that some patients practice another inflammatory disorder, hemophagocytic lymphohistiocytosis/macrophage activation syndrome (HLH/MAS), which is a toxicity mediated by abundant T-cell and macrophage activation and proliferation. Some of the laboratory abnormalities detected in patients treated with CAR T cells that suggest HLH/MAS include hyper-ferritinemia, coagulopathies, pancytopenia, and hem-ophagocytosis on bone marrow biopsies. 23-28 Whether this represents a separate inflammatory disorder from CRS or is potentially an overlap syndrome is difficult to distinguish based on the limited data on patient toxicities. Fortunately, because anti-inflammatory treatments such as corticosteroids are the mainstay of treatment for HLH/MAS, the distinction is not affecting management of clinical toxicity.
All clinical trials evaluating CD19-targeted CAR T cells for B-ALL have reported neurologic toxicities after treatment. These toxicities include word-finding difficulty, aphasia, encephalopathy, obtundation, and generalized seizures. 23-28 The exact mechanism for neurotoxicity is presently unknown. Neurotoxicities and CRS are considered to be separate toxicities because they can occur at disparate times during the clinical course. The UPENN group reported that six of thirteen cases of neurologic complications occurred after CRS had downright resolved. Twenty five However, neurologic toxicities are still very likely related to T-cell activation because similar complications develop in patients treated with blinatumomab. Forty two Indeed, CAR T cells can be detected in the cerebrospinal fluid after treatment, 23-27 and Turtle and colleagues twenty eight reported that peak serum cytokine levels correlated with severity of neurotoxicity. This suggests that en masse activation of the CAR T cells either directly or indirectly endows the cells with the capability to traverse the blood-brain barrier. However, the presence of B-ALL in the central jumpy system (CNS) also may contribute to neurologic toxicities because CAR T cells are enhanced in the CNS of patients with residual disease vs the CNS of patients without residual CNS disease. Severe (grade ≥3) neurotoxicity occurred in fifteen out of thirty patients in the FHCRC group and six of seventeen patients in the MSKCC group. 23,28 Neurotoxicity of any grade occurred in six of twenty one patients in the NCI group and thirteen of thirty patients in the UPENN group. 25,27
Management of neurotoxicity in these patients includes prophylaxis and medical interventions. Many patients received seizure prophylaxis medications, but presently there is no evidence that prophylaxis has diminished the number of neurologic complications and/or severity. As with CRS, the medical interventions for neurologic toxicities are tocilizumab and corticosteroids. 23-28 Albeit almost all patients ultimately react to corticosteroids, it is unclear whether tocilizumab ameliorates neurologic toxicities, given that this antibody is incapable to cross the blood-brain barrier. However, tocilizumab still may provide some benefit by reducing inflammation, which could impede the capability of CAR T cells to cross the blood-brain barrier. Fortunately, after the neurologic complications resolve, most patients have no long-term neurologic deficits except for partial amnesia regarding their hospital course.
The majority of CAR T cells evaluated for B-cell malignancies target CD19, which in addition to being voiced on most B-cell malignancies, is also voiced on normal B cells. As such, it was expected that a sturdy CAR T-cell response would also deplete normal B cells. Indeed, that was confirmed in early studies of patients with NHL who had prolonged B-cell aplasia that lasted a year or longer after CAR T-cell infusion. 17,Nineteen As more patients have been treated, there have been reports of B-cell aplasias lasting a year or longer. 23-28 These patients are managed with antibiotics and/or infusional gamma globulin until B cells recover. Presumably the lack of dangerous toxicities is related to the persistence of plasma cells that do not express CD19 and are able to secrete antibodies, thereby preserving humoral immunity. 43
In a brief time, CAR T cells have advanced from the bench to the bedside. The early-phase clinical trials exposed dramatic efficacy with durable remissions in patients with acute leukemias refractory to standard salvage chemotherapies. In the next duo of years, the very first gene-modified cell therapy will likely be approved, with an indication for B-cell malignancies. As CAR T-cell evaluation expands to multicenter phase two trials, the clinical expertise with this therapy will broaden. Other academic medical centers will mirror our institutions (Moffitt and Memorial Sloan Kettering), which have developed dedicated medical services to administer this therapy and manage the patients. With the ongoing development of numerous treatment sites, we expect further breakthroughs in understanding the nature of the toxicities and developing targeted supportive therapies that minimize the complications while preserving the therapeutic benefits. Furthermore, titillating preclinical work describing the next-generation of CAR T-cell therapeutics will hopefully permit this innovative, living drug to target not only B-cell malignancies, but other hematologic malignancies and solid tumors as well.
Dr Davila has no relevant conflict of interests to disclose. Dr Brentjens is a scientific cofounder and stockholder of Juno Therapeutics.
1. Dudley ME, Wunderlich JR, Shelton TE, Even J, Rosenberg SA. Generation of tumor-infiltrating lymphocyte cultures for use in adoptive transfer therapy for melanoma patients. J Immunother. 2003;26(Four):332-342.
Two. Rosenberg SA, Packard BS, Aebersold PM, et al. Use of tumor-infiltrating lymphocytes and interleukin-2 in the immunotherapy of patients with metastatic melanoma. A preliminary report. N Engl J Med. 1988;319(25):1676-1680.
Three. Jin J, Sabatino M, Somerville R, et al. Simplified method of the growth of human tumor infiltrating lymphocytes in gas-permeable flasks to numbers needed for patient treatment. J Immunother. 2012;35(Trio):283-292.
Four. Sadelain M, Brentjens R, Rivière I. The basic principles of chimeric antigen receptor design. Cancer Discov. 2013;Trio(Four):388-398.
Five. Hollyman D, Stefanski J, Przybylowski M, et al. Manufacturing validation of biologically functional T cells targeted to CD19 antigen for autologous adoptive cell therapy. J Immunother. 2009;32(Two):169-180.
6. Eshhar Z, Waks T, Gross G, Schindler DG. Specific activation and targeting of cytotoxic lymphocytes through chimeric single chains consisting of antibody-binding domains and the gamma or zeta subunits of the immunoglobulin and T-cell receptors. Proc Natl Acad Sci U S A. 1993;90(Two):720-724.
7. Sadelain M, Rivière I, Brentjens R. Targeting tumours with genetically enhanced T lymphocytes. Nat Rev Cancer. 2003;Trio(1):35-45.
8. Brocker T, Karjalainen K. Signals through T cell receptor-zeta chain alone are insufficient to prime resting T lymphocytes. J Exp Med. 1995;181(Five):1653-1659.
9. Finney HM, Lawson AD, Bebbington CR, Weir AN. Chimeric receptors providing both primary and costimulatory signaling in T cells from a single gene product. J Immunol. 1998;161(6):2791-2797.
Ten. Krause A, Guo HF, Latouche JB, Sunburn C, Cheung NK, Sadelain M. Antigen-dependent CD28 signaling selectively enhances survival and proliferation in genetically modified activated human primary T lymphocytes. J Exp Med. 1998;188(Four):619-626.
11. Kowolik CM, Topp MS, Gonzalez S, et al. CD28 costimulation provided through a CD19-specific chimeric antigen receptor enhances in vivo persistence and antitumor efficacy of adoptively transferred T cells. Cancer Res. 2006;66(22):10995-11004.
12. Brentjens RJ, Santos E, Nikhamin Y, et al. Genetically targeted T cells eradicate systemic acute lymphoblastic leukemia xenografts. Clin Cancer Res. 2007;13(Legitimate pt 1):5426-5435.
13. Imai C, Mihara K, Andreansky M, et al. Chimeric receptors with 4-1BB signaling capacity provoke potent cytotoxicity against acute lymphoblastic leukemia. Leukemia. 2004;Legitimate(Four):676-684.
14. Song DG, Ye Q, Poussin M, Harms GM, Figini M, Powell DJ Jr. CD27 costimulation augments the survival and antitumor activity of redirected human T cells in vivo. Blood. 2012;119(Three):696-706.
15. Savoldo B, Ramos CA, Liu E, et al. CD28 costimulation improves expansion and persistence of chimeric antigen receptor-modified T cells in lymphoma patients. J Clin Invest. 2011;121(Five):1822-1826.
16. Brentjens RJ, Rivière I, Park JH, et al. Safety and persistence of adoptively transferred autologous CD19-targeted T cells in patients with relapsed or chemotherapy refractory B-cell leukemias. Blood. 2011;118(Legitimate):4817-4828.
17. Kochenderfer JN, Wilson WH, Janik JE, et al. Eradication of B-lineage cells and regression of lymphoma in a patient treated with autologous T cells genetically engineered to recognize CD19. Blood. 2010;116(20):4099-4102.
Legitimate. Porter DL, Levine BL, Kalos M, Bagg A, June CH. Chimeric antigen receptor-modified T cells in chronic lymphoid leukemia. N Engl J Med. 2011;365(8):725-733.
Nineteen. Kalos M, Levine BL, Porter DL, et al. T cells with chimeric antigen receptors have potent antitumor effects and can establish memory in patients with advanced leukemia. Sci Transl Med. 2011;Trio(95):95ra73.
20. Kochenderfer JN, Dudley ME, Kassim SH, et al. Chemotherapy-refractory diffuse large B-cell lymphoma and indolent B-cell malignancies can be effectively treated with autologous T cells voicing an anti-CD19 chimeric antigen receptor. J Clin Oncol. 2015;33(6):540-549.
21. Kochenderfer JN, Dudley ME, Feldman SA, et al. B-cell depletion and remissions of malignancy along with cytokine-associated toxicity in a clinical trial of anti-CD19 chimeric-antigen-receptor-transduced T cells. Blood. 2012;119(12):2709-2720.
22. Porter DL, Hwang WT, Frey NV, et al. Chimeric antigen receptor T cells persist and induce sustained remissions in relapsed refractory chronic lymphocytic leukemia. Sci Transl Med. 2015;7(303):303ra139.
23. Davila ML, Riviere I, Wang X, et al. Efficacy and toxicity management of 19-28z CAR T cell therapy in B cell acute lymphoblastic leukemia. Sci Transl Med. 2014;6(224):224ra25.
24. Brentjens RJ, Davila ML, Riviere I, et al. CD19-targeted T cells rapidly induce molecular remissions in adults with chemotherapy-refractory acute lymphoblastic leukemia. Sci Transl Med. 2013;Five(177):177ra38.
25. Maude SL, Frey N, Shaw PA, et al. Chimeric antigen receptor T cells for sustained remissions in leukemia. N Engl J Med. 2014;371(16):1507-1517.
26. Grupp SA, Kalos M, Barrett D, et al. Chimeric antigen receptor-modified T cells for acute lymphoid leukemia. N Engl J Med. 2013;368(16):1509-1518.
27. Lee DW, Kochenderfer JN, Stetler-Stevenson M, et al. T cells voicing CD19 chimeric antigen receptors for acute lymphoblastic leukaemia in children and youthful adults: a phase one dose-escalation trial. Lancet. 2015;385(9967):517-528.
28. Turtle CJ, Hanafi LA, Berger C, et al. CD19 CAR-T cells of defined CD4+:CD8+ composition in adult B cell ALL patients. J Clin Invest. 2016;126(6):2123-2138.
29. Zola H, MacArdle PJ, Bradford T, Weedon H, Yasui H, Kurosawa Y. Prep and characterization of a chimeric CD19 monoclonal antibody. Immunol Cell Biol. 1991;Sixty-nine(Pt 6):411-422.
30. Brentjens RJ, Latouche JB, Santos E, et al. Eradication of systemic B-cell tumors by genetically targeted human T lymphocytes co-stimulated by CD80 and interleukin-15. Nat Med. 2003;9(Three):279-286.
31. Kochenderfer JN, Feldman SA, Zhao Y, et al. Construction and preclinical evaluation of an anti-CD19 chimeric antigen receptor. J Immunother. 2009;32(7):689-702.
32. Sommermeyer D, Hudecek M, Kosasih PL, et al. Chimeric antigen receptor-modified T cells derived from defined CD8+ and CD4+ subsets confer superior antitumor reactivity in vivo. Leukemia. 2016;30(Two):492-500.
33. Topp MS, Gökbuget N, Stein AS, et al. Safety and activity of blinatumomab for adult patients with relapsed or refractory B-precursor acute lymphoblastic leukaemia: a multicentre, single-arm, phase two explore. Lancet Oncol. 2015;16(1):57-66.
34. Thomas DA, Kantarjian H, Smith TL, et al. Primary refractory and relapsed adult acute lymphoblastic leukemia: characteristics, treatment results, and prognosis with salvage therapy. Cancer. 1999;86(7):1216-1230.
35. Zhao Z, Condomines M, van der Stegen SJ, et al. Structural Design of Engineered Costimulation Determines Tumor Rejection Kinetics and Persistence of CAR T Cells. Cancer Cell. 2015;28(Four):415-428.
36. Kawalekar OU, O’Connor RS, Fraietta JA, et al. Distinct Signaling of Coreceptors Regulates Specific Metabolism Pathways and Impacts Memory Development in CAR T Cells. Immunity. 2016;44(Two):380-390.
37. Sotillo E, Barrett DM, Black KL, et al. Convergence of Acquired Mutations and Alternative Splicing of CD19 Enables Resistance to CART-19 Immunotherapy. Cancer Discov. 2015;Five(12):1282-1295.
38. Gardner R, Wu D, Cherian S, et al. Acquisition of a CD19-negative myeloid phenotype permits immune escape of MLL-rearranged B-ALL from CD19 CAR-T-cell therapy. Blood. 2016;127(20):2406-2410.
39. Teachey DT, Rheingold SR, Maude SL, et al. Cytokine release syndrome after blinatumomab treatment related to abnormal macrophage activation and ameliorated with cytokine-directed therapy. Blood. 2013;121(26):5154-5157.
40. Suntharalingam G, Perry MR, Ward S, et al. Cytokine storm in a phase one trial of the anti-CD28 monoclonal antibody TGN1412. N Engl J Med. 2006;355(Ten):1018-1028.
41. Lee DW, Gardner R, Porter DL, et al. Current concepts in the diagnosis and management of cytokine release syndrome. Blood. 2014;124(Two):188-195.
42. Topp MS, Gökbuget N, Zugmaier G, et al. Phase II trial of the anti-CD19 bispecific T cell-engager blinatumomab shows hematologic and molecular remissions in patients with relapsed or refractory B-precursor acute lymphoblastic leukemia. J Clin Oncol. 2014;32(36):4134-4140.
43. Bhoj VG, Arhontoulis D, Wertheim G, et al. Persistence of long-lived plasma cells and humoral immunity in individuals responding to CD19-directed CAR T-cell therapy. Blood. 2016;128(Three):360-370.